Org. Synth. 1977, 56, 52
DOI: 10.15227/orgsyn.056.0052
ENONE REDUCTION-ENOLATE ALKYLATION SEQUENCE: 2-ALLYL-3-METHYLCYCLOHEXANONE
[Cyclohexanone, 3-methyl-2-(3-propenyl)-]
Submitted by Drury Caine
1, Sam T. Chao
1, and Homer A. Smith
2.
Checked by Mark E. Jason and Kenneth B. Wiberg.
1. Procedure
Caution! Since liquid ammonia is to be used, this preparation should be carried out in a well-ventilated hood.
A 2-l., three-necked, round-bottomed flask is fitted with a mechanical stirrer (Note 1), a pressure-equalizing dropping funnel, and a two-necked Claisen adapter holding a Dewar condenser (in the offset neck) and an adapter with stopcock for introduction of ammonia (Note 2). The entire apparatus is flame-dried under a stream of nitrogen, which enters at the top of the Dewar condenser, and a positive pressure of nitrogen is maintained throughout the experiment (Note 3). After anhydrous ammonia (1 l.) is introduced into the flask in liquid form (Note 4), the condenser is filled with an acetone–dry ice slurry, and the inlet adapter is removed and replaced with a glass stopper. Stirring is begun, and the ammonia is dried by adding very small pieces of lithium (ca. 5 mg. each) until the blue color persists (Note 5). Stirring is continued throughout the following reaction sequence.
Freshly-cut lithium wire (2.77 g., 0.400 g.-atom) (Note 6) is introduced into the flask and allowed to dissolve for 20 minutes, before a solution of 20.0 g. (0.182 mole) of 3-methyl-2-cyclohexen-1-one (Note 7) and 3.27 g. (0.182 mole) of water in 400 ml. of anhydrous diethyl ether (Note 8) and (Note 9) is added dropwise over 60 minutes (Note 10). Ten minutes after completion of the addition, a solution of 65 g. (0.54 mole) of allyl bromide (Note 11) in 150 ml. of anhydrous ether is added from the dropping funnel in a stream over 60 seconds (Note 12). Five minutes later 30 g. of ammonium chloride is added as rapidly as possible. Stirring is discontinued, the Dewar condenser is removed, and the ammonia is allowed to evaporate. A mixture of 300 ml. of ether and 300 ml. of water is added, and the reaction mixture is transferred to a separatory funnel and shaken. After separation of the ether layer, the aqueous layer is saturated with sodium chloride and extracted with two 100-ml. portions of ether. The combined ether extracts are washed with 100 ml. of 5% hydrochloric acid and 100 ml. of aqueous saturated sodium chloride and dried over anhydrous magnesium sulfate. After filtration, ether is removed with a rotary evaporator. The residual oil is distilled through a 37-cm. column packed with 6-mm. Raschig rings and equipped with a resistance heater for thermal balance. There is a forerun, b.p. 74–80° (12 mm.), consisting principally of 3-methylcyclohexanone, before 12.3–13.6 g. (45–49%) (Note 13) of 2-allyl-3-methylcyclohexanone distils as a 20:1 mixture of trans- and cis-isomers, b.p. 99–102° (12 mm.), nD24 1.4680–1.4683 (Note 14).
2. Notes
1.
A
glass stirring shaft and blade should be employed.
2.
An apparatus with four necks makes possible the introduction of solid reactants without removal of the condenser or dropping funnel.
3.
An apparatus similar to that described in
Org. Synth., Coll. Vol. 4, 132 (1963). was used for maintaining a
nitrogen atmosphere in the system. A positive
nitrogen flow was maintained whenever the system was open.
4.
Some commercial cylinders are provided with an
eductor tube, which allows the extraction of liquid
ammonia when the cylinder is upright. Cylinders not so equipped may be secured in a suitable
wooden or metal cradle, constructed so that the outlet valve is inclined below the body of the cylinder. The checkers dried commercial
ammonia with
sodium in a separate flask, then distilled the
ammonia into the reaction flask.
5.
Although water was used as a proton donor in this preparation, drying of the liquid
ammonia and
ether was carried out so that the lithium–ammonia solution would not be exposed to water during preparation and so that the amount of water added could be accurately controlled.
6.
Lithium wire, purchased from the Lithium Corporation of America, Inc., was cut in 2.5–5.0-cm pieces, washed free of the protective oil with ether, and weighed by transferring quickly to a
tared beaker containing mineral oil. The lengths of wire were again washed in a beaker of
ether and then held with forceps over an open neck of the reaction flask (positive
nitrogen flow), cut with scissors into 3–4-mm. pieces, and allowed to fall directly into the flask.
7.
3-Methyl-2-cyclohexen-1-one, b.p. 76–78° (11 mm.), was purchased from Aldrich Chemical Company, Inc., or prepared according to the procedure of Cronyn and Riesser.
3
8.
Ether was heated to reflux over
lithium aluminum hydride and distilled prior to use.
9.
The three-component mixture required a few minutes of shaking to ensure the formation of a homogeneous solution. It is essential that the water be completely dissolved before the solution is used.
10.
The deep blue solution of
lithium in liquid
ammonia generally turned pale blue at the end of the addition, and a white precipitate of the lithium enolate and/or
lithium hydroxide could be seen.
11.
Allyl bromide purchased from Eastman Organic Chemicals, Inc., was dried over
calcium chloride and distilled, b.p.
69–70° (760 mm.), prior to use.
12.
Since the
ammonia boils vigorously during this addition, it is advisable to open one neck of the flask to avoid excessive pressure buildup.
13.
The checkers obtained an additional
2–5 g. of
2-allyl-3-methylcyclohexanone by redistilling the column wash and the pot residue through a
short-path distillation column, raising the total yield to
14.9–18.1 g. (
54–66%).
14.
IR (neat) cm.
−1: 1714 (C=O), 1641 (C=C);
1H NMR (CCl
4), δ (number of protons, multiplicity): 0.82 and 1.10 (two d's, total of 3H, C
H3 of
cis- and
trans-isomers, respectively), 1.4–2.7 (m, 10H), 5.1 (two t's, total of 2H, -CH=C
H2), 5.8 (m, 1H, -C
H=CH
2). The distillate was analyzed by GC on a
3 mm. by 2 m. column containing 10% Carbowax 20M on HMDS Chromosorb W (60–80 mesh). Using a column temperature of 104° and a carrier gas flow rate of 30 ml./minute, the retention times for the
trans- and
cis-isomers were 9.0 minutes and 11.2 minutes, respectively. The product contained greater than 98% of the mixture of
trans- and cis-2-allyl-3-methylcyclohexanone.
A portion of the product was heated to reflux with
methanolic sodium methoxide to convert it into the thermodynamic mixture of
trans- (
ca. 65%) and
cis- (
ca. 35%) isomers. Small amounts of the isomers were collected by preparative GC using an
8 mm. by 1.7 m. column containing 15% Carbowax 20M on Chromosorb W, and each isomer exhibited the expected spectral and analytical properties. The same thermodynamic mixture of isomers was prepared independently by lithium–ammonia reduction
4 of
2-allyl-3-methylcyclo-2-hexen-1-one,
5 followed by equilibration with
methanolic sodium methoxide.
Using the
3 mm. by 2m. column described above, a mixture of stereoisomers of
2-allyl-5-methylcyclohexanone, prepared by allylation of the enamine of
3-methylcyclohexanone,
6 showed peaks at retention times of 8.4 minutes (more stable isomer) and 9.6 minutes. A mixture of the two isomeric 2-allyl-3-methylcyclohexanones and the two isomeric 2-ally-5-methylcyclohexanones clearly exhibited four distinct peaks.
3. Discussion
Alternative preparations of
2-allyl-3-methylcyclohexanone include (a)
lithium–ammonia reduction of
2-allyl-3-methyl-2-cyclohexen-1-one (see
(Note 13)), which can be prepared by alkylation of
3-methyl-2-cyclohexen-1-one or by alkylation of
ethyl 2-methyl-4-oxo-2-cyclohexene-1-carboxylate (Hagemann's ester), followed by hydrolysis and decarboxylation; and (b) conjugate addition of
lithium dimethylcuprate to
2-cyclohexen-1-one followed by trapping of the enolate with
allyl iodide7 or
allyl bromide8 in an appropriate solvent.
The present procedure utilizes the enone reduction–enolate alkylation procedure discovered by Stork and co-workers
9 and provides a means of directing alkylation to relatively inaccessible α-positions of unsymmetrical ketones. This procedure has been applied to the synthesis of specifically alkylated steroidal ketones,
10 decalones,
9 hydrindanones,
9 and monocyclic
4 and acyclic ketones.
11 The method is successful because, unlike enolates with other alkali metal counterions, lithium enolates of unsymmetrical ketones undergo alkylation with relatively reactive alkylating agents more rapidly then they equilibrate to isomeric enolates by proton transfer.
The direct conversion of
3-methyl-2-cyclohexen-1-one into
2-allyl-3-methylcyclohexanone provides an interesting example of the utility of the reduction-alkylation procedure. Synthesis of this compound from
3-methylcyclohexanone would be difficult because the latter is converted mainly into 2-alkyl-5-methylcyclohexanones either by direct base-catalyzed alkylation
12 or by indirect methods such as alkylation of its enamine (see
(Note 13)) or alkylation of the magnesium salt derived from its cyclohexylimine.
13
The procedure may also be extended to other alkylating agents and enones. We have reported
4 that
2,3-dimethylcyclohexanone can be prepared by reduction–methylation of
3-methyl-2-cyclohexen-1-one and have found that
2-benzyl-3-methylcyclohexanone is obtained by substituting
benzyl chloride for
allyl bromide in a procedure similar to that described above, but employing a longer alkylation time.
14 In a related case, Conia and Berlin
15 have prepared
3-(3-butenyl)-2-methylcyclohexanone by reduction–methylation of
3-(3-butenyl)-2-cyclohexen-1-one.
High-boiling products found in this procedure and in similar experiments involving cyclohex-2-enone derivatives
4 probably result from bimolecular reduction processes.
16,17 3-Methylcyclohexanone, which arises by protonation rather than alkylation of the enolate (and which made up
ca. 12% of the volatile products), is probably the result of reaction of
allyl bromide with liquid
ammonia to form the acidic species
allyl ammonium bromide.
4,11
Unless a proton donor is added, the lithium–ammonia reduction of an enone leads to the lithium enolate and lithium amide. The latter is a sufficiently strong base to rapidly convert the mono-alkylated ketone into its enolate (see
Figure 1), which can be further alkylated. The function of the added proton donor is to buffer the system by reaction with the
lithium amide.
tert-Butyl alcohol has been widely used as a proton donor in lithium–ammonia reductions,
4,9,11 but
lithium tert-butoxide is also a sufficiently strong base to cause a significant amount of polyalkylation in easily ionizable system. In a procedure similar to that above but in which
tert-butyl alcohol was substituted for water, and/or
2,2- 2,6-diallyl-3-methylcyclohexanone made up 15–20% of the volatile products. However, when water was used as the proton donor so that
lithium hydroxide was the strongest base present during the alkylation step, the amount of diallyl product was 2% or less.
Figure 1.
It is interesting that
trans-2-allyl-3-methylcyclohexanone is by far the major product of this reduction–alkylation sequence, being formed in greater than the equilibrium ratio (see
(Note 13)). The lithium enolate would be expected to exist in the two conformations shows in
Figure 2. The conformation having the methyl group quasiaxial may be quite important, because A
1,2-strain may be significant when the methyl group is quasiequatorial.
18 Recent work on the alkylation of lithium enolates of cyclohexanone derivatives has revealed that the stereochemistry of the reaction is governed largely by steric factors within the reactants.
19,20 From examination of models of the two conformations shown above, it appears that steric interactions between the methyl group and the approaching alkylating agent would be minimized in either of the two possible transition states which lead to the
trans product.
Figure 2.
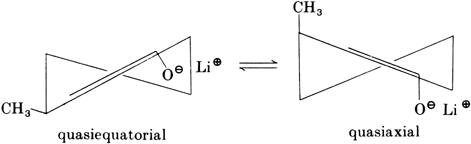
Appendix
Chemical Abstracts Nomenclature (Collective Index Number);
(Registry Number)
trans- and cis-2-allyl-3-methylcyclohexanone
2,2- 2,6-diallyl-3-methylcyclohexanone
calcium chloride (10043-52-4)
hydrochloric acid (7647-01-0)
ammonia (7664-41-7)
ether,
diethyl ether (60-29-7)
ammonium chloride (12125-02-9)
sodium chloride (7647-14-5)
Allyl bromide (106-95-6)
nitrogen (7727-37-9)
allyl iodide (556-56-9)
sodium methoxide (124-41-4)
sodium (13966-32-0)
benzyl chloride (100-44-7)
lithium (7439-93-2)
magnesium sulfate (7487-88-9)
lithium aluminum hydride (16853-85-3)
lithium amide (7782-89-0)
tert-butyl alcohol (75-65-0)
2-cyclohexen-1-one (930-68-7)
lithium hydroxide (1310-65-2)
3-methylcyclohexanone (591-24-2)
2-Allyl-3-methylcyclohexanone,
Cyclohexanone, 3-methyl-2-(3-propenyl)- (56620-95-2)
3-methyl-2-cyclohexen-1-one
2-allyl-5-methylcyclohexanone
2-allyl-3-methyl-2-cyclohexen-1-one,
2-allyl-3-methylcyclo-2-hexen-1-one
ethyl 2-methyl-4-oxo-2-cyclohexene-1-carboxylate
lithium dimethylcuprate
2,3-dimethylcyclohexanone
2-benzyl-3-methylcyclohexanone
3-(3-butenyl)-2-methylcyclohexanone
3-(3-butenyl)-2-cyclohexen-1-one
allyl ammonium bromide
lithium tert-butoxide (1907-33-1)
trans-2-allyl-3-methylcyclohexanone
Copyright © 1921-, Organic Syntheses, Inc. All Rights Reserved