Org. Synth. 2008, 85, 1
DOI: 10.15227/orgsyn.085.0001
ORGANOLITHIUMS AND LITHIUM 2,2,6,6-TETRAMETHYLPIPERIDIDE IN REDUCTIVE ALKYLATION OF EPOXIDES: SYNTHESIS OF (E)-ALKENES
[(E)-2-METHYLTETRADECA-1,3-DIENE]
Submitted by David M. Hodgson
1, Philip G. Humphreys, and Matthew J. Fleming.
Checked by Hiroyuki Morimoto, Hisashi Mihara, and Masakatsu Shibasaki.
1. Procedure
Caution! tert-Butylithium is extremely pyrophoric and must not be allowed to come into contact with the atmosphere. This reagent should only be handled by individuals trained in its proper and safe use. It is recommended that transfers be carried out by using a 20-mL or smaller glass syringe filled to no more than 2/3 capacity, or by cannula. For a discussion of procedures for handling air-sensitive reagents, see Aldrich Technical Bulletin AL-134. [Note added August 2009]
(E)-2-Methyltetradeca-1,3-diene. Caution! tert-Butyllithium is extremely pyrophoric and care must be taken to avoid exposure to air at all times. A 2-L, single-necked, round-bottomed flask (Note 1) is equipped with a magnetic stirrer bar and a rubber septum pierced by an argon inlet needle and an outlet needle. The flask, in which a positive flow of argon is maintained throughout the entire procedure, is flame-dried under argon, allowed to cool, and then charged by syringe with dry tetrahydrofuran (75 mL) (Note 2) and 2-bromopropene (6.24 mL, 70.2 mmol, 1.30 equiv) (Note 3). The solution is cooled to −78 °C (bath temperature) in a dry ice/acetone bath and then a 1.58 M solution of tert-butyllithium in pentane (89.2 mL, 140 mmol, 2.6 equiv) (Note 4) is added dropwise by syringe over 20 min in ten roughly equal portions (Note 5). The resulting bright-yellow suspension is stirred for 10 min at −78 °C, then an additional portion of 2-bromopropene (0.65 mL, 7.3 mmol, 0.14 equiv) is added dropwise over 7 min. The resulting pale-yellow suspension is stirred for an additional 30 min at −78 °C (during which time the preparation of the lithium 2,2,6,6-tetramethylpiperidide (LTMP) solution is initiated) and is warmed to 0 °C by transfer into an ice bath for 10 min. The resulting pale-yellow solution is re-cooled to −78 °C (bath temperature) (Note 6).
A 1-L, single-necked, round-bottomed flask is equipped with a magnetic stirrer bar and a rubber septum pierced by an argon inlet needle and an outlet needle. The flask, in which a positive flow of argon is maintained throughout the entire procedure, is flame-dried under argon, then is charged with dry pentanes (800 mL) (Note 7) and 2,2,6,6-tetramethylpiperidine (18.2 mL, 108 mmol, 2.0 equiv) by syringe (Note 8). The colorless solution is cooled to 0 °C in an ice bath and then a 1.57 M solution of n-butyllithium in hexanes (68.8 mL, 108 mmol, 2.0 equiv) (Note 4) is added dropwise by syringe over 15 min in seven roughly equal portions (Note 9). The resulting yellow LTMP solution is stirred at 0 °C for 30 min further and then is added via cannula (Note 10) over 15 min to the stirring pale-yellow suspension of 2-propenyllithium at −78 °C (Note 11). The resulting yellow suspension is transferred to an ice bath, is stirred for 30 min and then 1,2-epoxydodecane (11.8 mL, 54.0 mmol) (Note 8) is added dropwise by syringe in three roughly equal portions over 5 min. The ice bath is removed and the resulting yellow suspension is stirred for 2 h (Note 12).
The bright-yellow solution is re-cooled to 0 °C and 50 mL of a 1 M aqueous hydrochloric acid solution is added by syringe over 5 min (Note 13). The reaction mixture is poured into a 3-L separatory funnel containing 1 M aqueous hydrochloric acid solution (200 mL). The reaction flask is rinsed with diethyl ether (2 × 100 mL), which is added to the separatory funnel. After thorough mixing, the aqueous layer is separated and extracted with diethyl ether (3 × 200 mL). The combined organic extracts are washed with brine (200 mL), dried over magnesium sulfate, filtered and evaporated under gradually reduced pressure (40 °C, 760 mmHg to 15 mmHg) using a rotary evaporator to leave 18.6 g of a pale-yellow residue (Note 14).
The yellow residue is loaded neat onto a pad of silica gel (15 cm × 6 cm) (Note 15) that has already been wetted with n-pentane, and the flask is rinsed with pentane (2 × 10 mL) (Note 16). The pad is rinsed with 1000 mL of n-pentane and the eluent is collected directly as a single fraction. Rotary evaporation under reduced pressure (40 °C, 760 mmHg to 15 mmHg) for 2 h (Note 17) gives 6.47 g (58%) (Note 18) of (E)-2-methyltetradeca-1,3-diene as a colorless oil (Note 19). The E/Z ratio of this product is determined to be 97:3 (Note 20).
2. Notes
1.
All apparatus was oven-dried overnight.
2.
The submitters purchased
tetrahydrofuran (99+%) from Rathburn Chemicals Ltd. and the solvent was freshly distilled from sodium and benzophenone under an atmosphere of nitrogen. The checkers purchased
tetrahydrofuran (anhydrous, 99.9%, inhibitor free) from Aldrich Chemical Co. and freshly distilled the solvent from sodium and benzophenone under an atmosphere of argon. The checkers determined the amount of water in the distilled tetrahydrofuran to be 4.0 ppm by Karl-Fischer titration.
3.
2-Bromopropene (99%) was purchased from Aldrich Chemical Co. The submitters used it as received. The checkers distilled it (bp 50-51 °C) from CaCl
2 under an atmosphere of argon before use.
4.
The submitters purchased
tert-butyllithium (1.5 M in pentane) and n-butyllithium (1.6 M in hexanes) from Fisher Scientific Worldwide Co. Checkers purchased
tert-butyllithium (1.57 M in n-pentane) from Kanto Chemical Co. and
n-butyllithium (1.6 M in hexanes) from Aldrich Chemical Co., respectively. The organolithium reagents were used as received. Fresh 100-mL bottles of the organolithiums were used for each reaction run. The solutions were titrated prior to use using
s-BuOH and 1,10-phenanthroline according to an established procedure.
2
5.
tert-Butyllithium formed a bright yellow complex with tetrahydrofuran at −78 °C, whereas 2-propenyllithium was colorless. If a bright (rather than pale) yellow color persisted following the addition of
tert-butyllithium, the excess
tert-butyllithium was consumed by slow addition of additional
2-bromopropene (0.65 mL by the checker, a few drops by the submitter) until a pale yellow/colorless solution remained.
3 When the bright yellow solution was used directly, the product was obtained in lower purity with a detectable amount of by-products (presumably derived from the excess
tert-butyllithium). Addition rate of
tert-butyllithium (within 20 min in a full scale and within 10 min in a half scale) is also important to obtain reproducible results. When the checkers added
tert-butyllithium slowly over 50 min, the product was obtained in lower yield and purity.
6.
At this concentration 2-propenyllithium was a solution in THF at 0 °C and a suspension at −78 °C.
7.
To prevent precipitation of LTMP,
800 mL of pentane was required.
Pentane was purchased by the submitters from Rathburn Chemicals Ltd. and was degassed with nitrogen and passed through alumina under an atmosphere of nitrogen directly into the pre-calibrated flask.
4 The checkers purchased
pentane (anhydrous, 99+%) from Aldrich Chemical Co. and used it as received. The checkers determined the amount of water in the pentane to be 6.0 ppm by Karl-Fischer titration.
8.
2,2,6,6-Tetramethylpiperidine (99%) was purchased from Aldrich Chemical Co. 1,2-Epoxydodecane (95%) was purchased from Aldrich Chemical Co. 2,2,6,6-Tetramethylpiperidine was distilled from CaH2 under an atmosphere of argon (bp 150-152 °C) and 1,2-epoxydodecane was distilled from CaH
2 under reduced pressure (bp 110-112 °C, 7.5 mmHg).
9.
Use of 2.0 equiv of LTMP was necessary to minimize by-product formation. Carrying out the reaction with 1.3 equivalents of LTMP gave a 37% yield of (
E)-2-methyltetradeca-1,3-diene, a 5% yield of dodecanal
5 and a 41% yield of 2-methyltetradec-1-en-4-ol. If LTMP was omitted from the reaction, 2-methyltetradec-1-en-4-ol was isolated in 96% yield.
10.
An oven-dried, 14 gauge cannula was used.
11.
A bleed needle was used in the flask containing the 2-propenyllithium suspension to allow transfer to occur rapidly.
12.
The reaction was monitored by TLC analysis on aluminum-backed plates pre-coated with silica containing a fluorescent indicator (0.2 mm, Merck 60 F
254, purchased from Merck KGAA) using
n-pentane/diethyl ether (20:1) as the eluent.
The plates were visualized following staining with basic potassium permanganate solution and gentle heating. The product diene had R
f = 0.96 (weakly UV active; bright yellow after staining); the epoxide starting material had R
f = 0.38 (UV inactive; white to yellow); dodecanal had R
f = 0.35 (UV inactive; white to yellow) and 2-methyltetradec-1-en-4-ol had R
f = 0.12 (UV inactive; yellow).
13.
Although the submitters observed evolution of gas, the checkers did not observe significant evolution of gas.
14.
It was important to remove solvent well at this stage to avoid separation problems during silica gel column chromatography.
15.
Silica gel (Kieselgel 60) was purchased from Aldrich Chemical Co.
16.
n-Pentane (>98% purity) was purchased from Wako Chemical Co. and used as received. The submitters used light petroleum ether (30-40 °C, 99+%) from Rathburn Chemicals Ltd. The checkers found that the use of normal petroleum ether (b.p. 30-60 °C) caused problems due to incomplete removal of petroleum ether residue at the last stage of evaporation.
17.
The control of pressure during the evaporation (20 kPa) was important to remove
n-pentane: when evaporation was performed at 27 kPa for 2 h, the residual
n-pentane was observed in
1H and
13C NMR spectra of the product.
18.
Submitters obtained the product in 65% yield. Checkers obtained the product in 58% yield on a half-scale reaction. Purity of the product was >95% by gas chromatographic analysis using a 30 m, 0.25 mm internal diameter, 95% dimethyl-5% diphenylpolysiloxane capillary column (
tR = 10.5 min, initial temp. 200 °C, column temp. 150 °C, detection temp. 250 °C). A minor component (presumably the
Z-isomer) was detected at
tR = 8.6 min (1.4 %). If necessary, the product can be further purified by distillation under reduced pressure (9 mmHg, bp 120-121 °C, >95% purity by GC analysis).
19.
The diene had the following characteristics: bp 120-121 °C, 9 mmHg; IR (neat) 3082 (m), 3015 (m), 2925 (s), 2853 (s,) 1767 (w), 1647 (w), 1609 (m), 1456 (s), 1377 (m), 1313 (w), 1085 (w), 964 (s), 881 (s), 721 (m) cm
−1;
1H NMR
pdf (500 MHz, CDCl
3) δ: 0.89 (t,
J = 6.7 Hz, 3 H), 1.21-1.35 (m, 14 H), 1.35-1.45 (m, 2 H), 1.84 (brs, 3 H), 2.10 (brtd,
J = 7.0, 7.0 Hz, 2 H), 4.86 (brs, 2 H), 5.67 (td,
J = 7.0, 15.6 Hz, 1 H), 6.14 (brd,
J = 15.6 Hz, 1 H);
13C NMR
pdf (125 MHz, CDCl
3) δ: 14.1, 18.7, 22.7, 29.3, 29.4, 29.5, 29.5, 29.6, 29.6, 31.9, 32.8, 114.0, 131.1, 132.7, 142.2; LRMS (EI)
m/z 208 (M
+); HRMS (EI)
m/z calcd for C
15H
28+ (M
+), 208.2191, found 208.2190. Anal. Calcd for C
15H
28: C, 86.46; H, 13.54. Found: C, 86.06; H, 13.75.
20.
The
E/
Z ratio determined by NMR analysis [
1H NMR (500 MHz, CDCl
3); minor
Z isomer: δ: 2.20-2.31 (m, 2H, 5-C
H2), major
E isomer: δ: 2.10 (brtd,
J = 7.0, 7.0 Hz, 2 H, 5-C
H2).
3. Discussion
The ability to prepare carbon-carbon double bonds in a regio- and stereocontrolled manner is of central importance in organic synthesis. One such method originally reported by Crandall and Lin,
6 and subsequently studied in more detail by Mioskowski and co-workers,
7 involves addition of an organolithium to an epoxide to give the corresponding alkene. However, the reaction in its original manifestation suffers from three significant limitations: (1) only simple alkyllithiums are effective partners; (2) high
E-selectivity is observed only with secondary and tertiary alkyllithiums; and (3) at least 2 equiv of the organolithium are required.
The LTMP-modified reductive alkylation of epoxides reported herein provides a solution to the limitations mentioned above allowing rapid, convergent, and position-specific access to alkenes in high
E/
Z purity from readily available starting materials (Table 1).
8
Table 1. Alkenes from epoxides using LTMP and organolithiums.a
a Reactions performed on 0.43-1.25 mmol scale. b Isolated yield. c Determined by GCMS. Ratios refer to E:Z (entries 1-4, 11, 12), E,E:Z,E (entries 5-7, 10), Z,E:E,E (entry 8) and Z,E:other isomers (entry 9). d Et2O as solvent (in hexane 84% yield, E:Z, 65:35). e 1.3 equiv of LTMP used.
The use of alkenyllithiums gives the corresponding diene in high isomeric purity, while employing aryllithiums gives arylated alkenes.
E-Allylsilanes, which are valuable intermediates in synthesis,
9 are also conveniently prepared by addition of a terminal epoxide to a mixture of LTMP and an a-silyl-organolithium.
We propose that LTMP functions as a base to form trans-a-lithiated epoxide 1, then the organolithium can act as a nucleophile on this transient carbenoid (Scheme 1). Subsequent elimination of Li2O gives the observed alkenes in generally high isomeric purity.
Scheme 1
Addition of the organolithium reagent to the terminal epoxide in the absence of LTMP gives the corresponding secondary alcohol from direct ring-opening of the epoxide as the major product.
Appendix
Chemical Abstracts Nomenclature (Collective Index Number);
(Registry Number)
2-Bromopropene; (557-93-7)
tert-Butyllithium: Lithium, (1,1-dimethylethyl)-; (5944-19-4)
n-Butyllithium: Butyllithium; (109-72-8)
2,2,6,6-Tetramethylpiperidine: (768-66-1)
1,2-Epoxydodecane: Oxirane, decyl-; (2855-19-8)
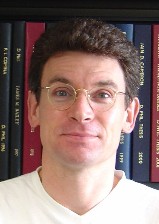 |
David Hodgson obtained his first degree in Chemistry at Bath University. After a PhD at Southampton University in the field of natural product synthesis (with P. J. Parsons) and a research position at Schering, he was appointed in 1990 to a lectureship at Reading University. In 1995 he moved to the Chemistry Department at Oxford University, where he is now a Professor of Chemistry. His research interests are broadly in the development and application of synthetic methods, particularly (asymmetric) generation and transformations of carbenoids. |
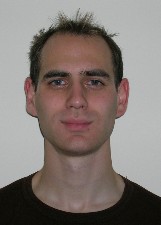 |
Philip Humphreys was born in 1981 in Worcester, UK. After obtaining his MSci in Biochemistry and Biological Chemistry (first class honors) in 2003 from the University of Nottingham, he moved to the University of Oxford (Oriel College) for his PhD studies under the supervision of David Hodgson. His work was sponsored by GlaxoSmithKline and concerned the reactions of alpha-lithiated aziridines. Phil is currently a postdoctoral researcher with Larry Overman at UC Irvine, supported by a Merck Sharp & Dohme Postdoctoral Research Fellowship. |
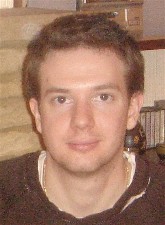 |
Matthew Fleming was born in 1978 in London, UK. After obtaining his MSci in Chemistry (1st class honors) in 2002 from King's College London, he moved to the University of Oxford (Hertford College) and in 2005 completed his PhD under the supervision of David M. Hodgson. His research was sponsored by GlaxoSmithKline and was concerned with the development of new reaction methodology involving metalated heterocycles and sulfur ylides. He is currently a postdoctoral research assistant with Prof. Mark Lautens at the University of Toronto, working in the area of natural product synthesis. |
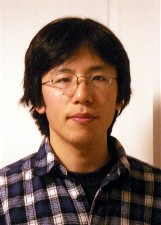 |
Hiroyuki Morimoto was born in 1981 in Hiroshima, Japan. He graduated in 2004 and received his M. S. degree in 2006 from the University of Tokyo under the direction of Professor Masakatsu Shibasaki. The same year he started his Ph. D. study under the supervision of Professor Shibasaki. His current interest is development of novel direct asymmetric catalytic systems using ester equivalents as donor substrates. |
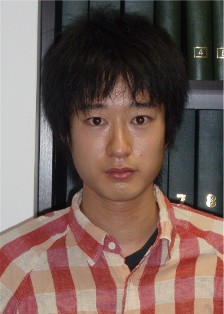 |
Hisashi Mihara was born in 1981 in Shiga, Japan. He received his M. S. degree in 2006 from the University of Tokyo. He is pursuing Ph.D. degree at the Graduate School of Pharmaceutical Sciences, The University of Tokyo, under the guidance of Professor Masakatsu Shibasaki. His current interest is catalytic enantioselective total synthesis of biologically active compounds. |
Copyright © 1921-, Organic Syntheses, Inc. All Rights Reserved