Org. Synth. 2012, 89, 334-349
DOI: 10.15227/orgsyn.089.0334
Discussion Addendum for:
[Bicyclopropylidene]
Submitted by Armin de Meijere* and Sergei I. Kozhushkov
1.
Discussion
This facile and easily scalable synthesis
2 has made bicyclopropylidene (BCP), a uniquely strained, tetrasubstituted alkene, accessible in bulk quantities.
Thus, various applications of BCP as a multifunctional C
6 building block, and in mechanistic terms, for the understanding of certain reaction principles,
3 have been developed over the past decade.
Preparation and Functionalization
The method shown above has been extended to the preparation of ring-annelated BCPs.
4 The best approach to functionally monosubstituted BCPs is by deprotonation with
n-butyllithium in THF at 0 °C followed by electrophilic substitution of the lithiobicyclopropylidene with appropriate reagents.
5 Some recent examples
6-8 are shown in Figure 1, while the previously published ones are summarized in reviews.
3b,c
Figure 1.
Functionalization of BCP.
6-8
On the other hand, oligosubstituted, as well as oligospirocyclo-propanated BCPs, can better be prepared according to Neuenschwander et al.
9 by dehalogenative coupling of
1-halo-1-lithiocyclopropanes generated from
1,1-dihalocyclopropanes upon treatment with alkyllithium reagents in the presence of copper(II) salts (see below).
Chemical Transformations
I.
Isomerizations
BCP undergoes a clean rearrangement to
methylenespiropentane when passed through a hot tube at 350 °C.
3a,c,e In view of the ready availability of BCP, this rearrangement constitutes the most convenient preparative approach to methylenespiropentane.
8 When heated in a closed vessel as a pure compound or in solution (50% in toluene), a substantial fraction of BCP dimerizes to yield [4]rotane.
10 Theoretical aspects of the thermal,
3e,8,11 as well as the photochemical,
12 isomerizations of BCP have also been studied in detail.
II.
Additions onto the Double Bond
While bromine addition to BCP and spirocyclopropanated BCPs is mainly of theoretical importance,
13 hydrogenation of substituted BCPs has been used several times to prepare specific compounds.
Thus, a series of ω-
trans-(bicyclopropyl)-substituted fatty acids for biological studies has been synthesized from BCP.
14 The key step in these preparations was a
Figure 2.
Reduction of the double bond in BCPs to yield bicyclopropyls.
14
reasonably diastereoselective Birch reduction of the bicyclopropylidene moiety with lithium in liquid ammonia to afford THP-protected
trans-(bicyclopropyl)alkanol derivatives in 63-88% isolated yields (Figure 2).
2-(trans-2'-Cyclopropylcyclopropyl)-4,4,5,5-tetramethyl-1,3-dioxa-2-borolane - an important intermediate for palladium-catalyzed cross-coupling reactions - was also diastereoselectively prepared in 79% yield applying such a Birch reduction (Figure 2).
6
On the other hand, hydrogenation of bicyclopropylidenes with diimide, in situ generated from 2-nitrobenzenesulfonyl hydrazide, furnished predominantly
cis-bicyclopropyl derivatives.
On the basis of these two reduction methods, five of the six possible diastereomeric [1,1';2',1'';2'',1''']quatercyclopropanes were obtained from diastereomeric
meso- and
d,
l-bis(bicyclopropylidenyl).
15
1-Cyclopropylcyclopropaneboronate
- a starting material for the synthesis of derivatives of the skeletally interesting [1,1';1',1'';1'',1''']quater- and [1,1';1',1'';1'',1''';1''',1''''] quinquecyclopropan-1-ol by a Matteson-type homologation
16 on
dibromocyclopropane
- could be obtained from the hydroboration product of BCP, yet, in only 27% yield (Figure 3).
17
Figure 3.
Hydroboration of the double bond in BCP and application of the product in the preparation of [1,1';1',1'';1'',1''']quater- and [1,1';1',1'';1'',-1''';1''',1'''']quinquecyclopropanol.
17a
Interestingly, the 1,1-linked cyclopropane moieties in dinitrobenzoates of these alcohols in the crystals adopt helical
all-
gauche conformations.
17a Such helical overall structures have also been proved for higher 1,1-linked oligocyclopropyl hydrocarbons, which have consequently been termed [
n]ivyanes
17b,c by Sherburn et al.
17b
III.
Cycloadditions.
Preparation of Triangulanes and Heterotriang-ulanes
Among other purposes, BCP serves as the best starting material for various linear and branched [
n]triangulanes - hydrocarbons consisting exclusively of spiroannelated cyclopropane units.
3f,18 Thus, along with the well-known 7,7-dibromo[3]triangulane,
3a-c a new large family of 7-substituted [3]triangulanes (dispiro[2.0.2.1]heptanes) has been prepared during the last decade by halocarbenoid transfer to BCP under different conditions (Figure 4).
19,20 Among the chemical transformations of such [3]triangulanes, relative stabilities of spirocyclopropanated cyclopropyl cation intermediates in solvolytic processes
20 and unusual rearrangements of dibromotriangulanes in their reactions with alkyllithiums
19,21,22 have been studied in especially great detail.
Figure 4.
Addition of dihalocarbenes onto BCP.
19-21
On the other hand, 7,7-dibromo[3]triangulane can be subjected to dehalogenative coupling under the conditions of Neuenschwander et al.
9 in a repetitive fashion to afford perspirocyclopropanated bicyclopropylidenes.
Along this route, the record-setting
C2v-[15]triangulane with 15 spirofused cyclopropane rings was built up starting from BCP.
23 An analogous key step - the dehalogenative coupling of a dibromocyclopropane to a bicyclopropylidene - was employed in the syntheses of enantiomerically pure linear [7]-, [9]- and [15]triangulanes,
24 while enantiomerically pure linear [4]- and [5]triangulanes were prepared from BCP itself.
25
Epoxidations,
26 episulfidation,
27 reaction with a stable dialkylsilylene,
28 and CuCl-catalyzed phosphinidene addition
29 to BCP and spirocyclopropanated BCPs as well as their complexation
30 yielded 7-oxa-, 7-thia-, 7-sila-, and 7-phospha-, and 7-metalla[3]triangulane, respectively, as well as their spirocyclopropanated analogues (Figure 5).
Figure 5.
Hetera- and metallatriangulanes prepared from BCP during the last decade.
While BCP did not react with a photolytically generated diethynylcarbene,
31 it furnished ethyl 7-nitrodispiro[2.0.2.1]heptane-7-carboxylate with the rhodium carbenoid generated from ethyl nitrodiazoacetate, yet in only 13% yield.
32 The yield of the successful cyclopropanation with diazoacetonitrile has not been reported.
33
IV.
1,3-Dipolar Cycloadditions of Nitrones
The Brandi-Guarna reaction, i.
e.
the stereo- and regioselective thermal rearrangement of spirocyclopropanated isoxazolidines and isoxazolines obtained by 1,3-dipolar cycloadditions of nitrones and nitrile oxides to alkylidenecyclopropanes into tetrahydro- or dihydropyridones (Figure 6),
34 in its extension to BCP yielded a number of spirocyclopropanated heterocyclic ketones.
3b,c Some of these products have
Figure 6.
1,3-Dipolar cycloadditions of nitrones to BCP and thermal rearrangement of the products.
35-39
turned out to cleave supercoiled DNA in analogy to certain cytotoxic natural products like the illudines and ptaquiloside.
At least 15 new functionally substituted bisspirocyclopropanated isoxazolidines have been prepared from BCP in good overall yields (average 83%) (Figure 6)
35-38 and employed in e.
g.
thermal rearrangements to afford spirocyclopropane-annelated tetrahydropyridones.
36,37,39 In the case of
N-arylisoxazolidines, spirocyclopropanated benzoazocinones were formed in moderate yields (21-27%).
39
Chemoselective reduction of the N-O bond of the isoxazolidine ring in bisspirocyclopropanated isoxazolidines afforded substituted 1'-(aminomethyl)bi(cyclopropan)-1-ols (70-90% yield), which underwent a Pd(II)-catalyzed cascade rearrangement into a 1:1 mixture of 3-spirocyclopropanedihydro- and -tetrahydropyrid-4-ones (Figure 7).
36 Under the influence of trifluoroacetic acid in acetonitrile at 70 °C, these bisspirocyclopropanated isoxazolidines undergo a fragmentative thermal rearrangement to furnish the corresponding 3-spirocyclopropanated β-lactams in 75-96% yields (Figure 7).
38 Some such β-lactams could also be
Figure 7.
Transformations of bisspirocyclopropanated isoxazolidines.
35,36,38,40-42
synthesized in a one-pot three-component reaction upon microwave heating of mixtures of BCP, certain alkylhydroxylamine hydrochlorides and formaldehyde (or an alkyl glyoxylate) in the presence of sodium acetate in ethanol for 15-120 min in 49-78% yields (Figure 7).
40
The acid-catalyzed fragmentative thermal rearrangement of some bicyclic bisspirocyclopropanated isoxazolidines was accompanied by an opening of the β-lactam ring affording protected derivatives of α-cyclopropyl-β-homoproline (Figure 7).
35 The latter were used for the preparation of biologically active pseudotripeptides (Figure 7).
At last, all the transformations discussed above have been realized in their intramolecular versions as well.
41,42
V.
Additions and Cycloadditions in the Presence of Transition Metals
Metal-mediated or -catalyzed reactions of methylenecyclopropanes including BCP have been studied extensively and found to proceed almost exclusively with opening of a cyclopropane ring,
43 whereas cyclopropane-retained compounds were observed as side products at best.
In contrast to this, conservation of both rings was observed in the first ruthenium-catalyzed intermolecular hydroarylation of BCP via C-H bond functionalization, a remarkable reaction mode for a transformation proceeding through a (cyclopropylcarbinyl)metal intermediate (Figure 8).
44
Figure 8.
Metal-catalyzed additions onto the double bond in BCP.
44,45
On the other hand, inter- and intramolecular palladium-catalyzed additions of disilanes, silylboranes, silylstannanes, and silyl cyanides across the double bond of BCP can proceed in two modes - with and without opening of one cyclopropane ring - yielding either bicyclopropyl or cyclopropylidenepropane derivatives depending on the nature of the reagent and the palladium catalyst (Figure 8).
45
The carbonyl ylide 1,3-dipoles, generated by dirhodium tetraacetate-catalyzed decomposition of 1-diazo-5-phenylpentane-2,5-dione and 1-(1-acetylcyclopropyl)-2-diazoethanone, cycloadd to BCP to give bridgehead-substituted di- and trispirocyclopropanated 8-oxabicyclo[3.2.1]octan-2-one and 7-oxabicyclo[2.2.1]heptan-2-one, respectively, yet only in 57 and 16% yield (Figure 9).
46 Intramolecular Pauson-Khand reactions of BCP derivatives
47 and cobalt(I)-mediated intramolecular [2+2+2] cocyclizations of (bicyclopropylidenyl)diynes
48 also proceeded with retention of both
Figure 9.
Cocyclizations of BCPs in the presence of transition metals with conservation of both cyclopropane rings.
46
cyclopropane rings and afforded cyclopropane-fused and spirocyc-lopropanated bicyclo[3.3.0]alkenones)
47 or cobalt-complexed tricyclo-[7.3.0.0
2,6]dodeca-1,6-dienes,
48 respectively, in moderate to good yields.
Figure 10.
[4+1] Cocyclization of BCP with Fischer carbenechromium complexes and nickel-catalyzed intermolecular [3+2+2] cocyclizations of BCP and alkynes.
49,50
![Figure 10.
[4+1] Cocyclization of BCP with Fischer carbenechromium complexes and nickel-catalyzed intermolecular [3+2+2] cocyclizations of BCP and alkynes.Kurahashi, T.; Wu, Y.-T.; Meindl, K.; Rühl, S.; de Meijere, A.
Synlett 2005, 805-808.,Zhao, L.; de Meijere, A.
Adv.
Synth.
Cat.
2006, 348, 2484-2492.](/content/figures/V89P0334-11.gif)
On the other hand, Fischer carbenechromium complexes react with BCP in an unprecedented manner: All four carbon atoms of one of the methylenecyclopropane moieties of BCP along with carbon monoxide are incorporated with the formation of three new C-C-bonds to give substituted cyclopentenone derivatives in good yields (65-72%) (Figure 10).
49 Under the catalysis of bis(1,5-cyclooctadiene)nickel(0), in the presence of triphenylphosphine, two molecules of BCP and one molecule of a terminal alkyne at ambient temperature, undergo an intermolecular [3+2+2] cocyclization with opening of one cyclopropane ring to furnish bis-spirocyclopropanated cyclopropylidenecycloheptene derivatives in 45-93% yields (Figure 10).
50 For comparison, methylenecyclopropanes under these conditions experience an intermolecular [3+2+2] cocyclization of one molecule of methylenecyclopropane and two molecules of a terminal alkyne.
51
VI. Domino Heck-Diels-Alder Reactions
The previously reported Heck coupling of haloarenes and BCP afforded 1-arylallylidenecyclopropanes, which can be trapped in a Diels-Alder reaction with an appropriate dienophile to give 8-arylspiro[2.5]octenes (Figure 11).
52,53 In the presence of trisfurylphosphine instead of triphenylphosphine the 1-arylallylidenecyclopropanes undergo readditions of the hydridopalladium halide to yield s-allylpalladium intermediates, which are efficiently trapped with various nitrogen and carbon nucleophiles
Figure 11.
Two reaction modes of BCP under Heck conditions and domino Heck-Diels-Alder reactions.
52-56
to yield functionally substituted methylenecyclopropane derivatives (Figure 11).
53,54 Both these reaction modes were further developed during the last decade.
Thus, a new large family of substituted spiro[2.5]oct-4-ene derivatives has been synthesized from BCP employing this highly efficient three-component domino reaction, in most cases in good to very high yields (49-100%) (Figure 11).
55 Moreover, the coupling of oligoiodobenzenes with BCP and subsequent cycloaddition was extended to this multicomponent reaction.
For example, 1,2,4,5-tetraiodobenzene gave the fourfold domino Heck-Diels-Alder product in 47% isolated yield, in a single operation, in which 12 new carbon-carbon bonds were formed.
55
This three-component domino reaction has also been extended to monosubstituted BCPs.
56 The second reaction mode of BCP with an intermediate s-allylpalladium species in the presence of a secondary amine and trisfurylphosphine was further developed into a one-pot, four-component two-step queuing cascade with iodoethenes and secondary amines to provide 8-(10-aminoethyl)-substituted spiro[2.5]oct-7-ene derivatives (Figure 12).
57 The same one-pot, two-step queuing cascade can be carried out with cyclic dienophiles such as
N-arylmaleinamides and
N-phenyltriazolinedione to furnish highly substituted spiro[2.5]oct-4-enes and spirocyclopropanated heterobicycles.
Figure 12.
Four-component two-step queuing cascade reactions of BCP and palladium-catalyzed cross-coupling/electrocyclization cascade reactions.
57-59
Finally, further cascade reactions involving Heck-type coupling of BCP with 2-bromo-1,6-enynes afforded cross-conjugated tetraenes which, at elevated temperatures, underwent 6p-electrocyclization to give spiro[cyclopropane-1,4'-bicylo[4.3.0]-1(6),2-dienes] (Figure 12).
58,59
Appendix
Chemical Abstracts Nomenclature (Collective Index Number);
(Registry Number)
Dibromocyclopropane
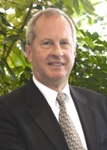 |
Armin de Meijere, born 1939, studied chemistry in Freiburg and Göttingen, receiving a doctoral degree (Dr.
rer.
nat.) in 1966 in Göttingen, completing postdoctoral training at Yale University in 1967-1969, and receiving Habilitation in 1971 in Göttingen.
He was appointed full professor of organic chemistry in Hamburg, 1977-1989 and ever since has been in Göttingen.
He was visiting professor at 16 research institutions around the world including the University of Wisconsin, the Technion in Haifa, Israel, and Princeton University.
His awards and honors include member of the Norwegian Academy of Sciences, Alexander von Humboldt/Gay Lussac prize, Honorary Professor of St.
Petersburg State University in Russia, Adolf von Baeyer Medal of the German Chemical Society, Dr.
honoris causa of the Russian Academy of Sciences.
He has been or still is Editor or member of the editorial board of a number of scientific publications including Houben-Weyl, Chemical Reviews, Science of Synthesis, and Chemistry - A European Journal.
His scientific achievements have been published in over 700 original publications, review articles, and book chapters as well as 25 patents.
|
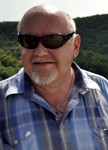 |
Sergei I.
Kozhushkov was born in 1956 in Kharkov, USSR.
He studied chemistry at Lomonosov Moscow State University, where he obtained his doctoral degree in 1983 under the supervision of Professor N.
S.
Zefirov and performed his "Habilitation" in 1998.
From 1983 to 1991, he worked at Moscow State University and then at Zelinsky Institute of Organic Chemistry.
In 1991, he joined the research group of Professor A.
de Meijere (Georg-August-Universität Göttingen, Germany) as an Alexander von Humboldt Research Fellow; since 1993 he has worked as a Research Associate, since 1996 he has held a position of a Scientific Assistant, and since 2001 a permanent position as a Senior Scientist at the University of Göttingen.
His current research interests focus on the chemistry of highly strained small ring compounds.
The results of his scientific activity have been published in over 200 original publications, review articles, book chapters and patents.
From 2003-2011, he won eight awards from InnoCentive, Inc.
|
Copyright © 1921-, Organic Syntheses, Inc. All Rights Reserved